Particle Dynamics Simulation
Simulation of Particles in Electromagnetic Fields
Vacuum Electron Devices, Particle Accelerators and More
The SIMULIA simulation portfolio comprises a wide range of solvers, enabling the simulation of devices that operate employing the interaction of free-moving particles and electromagnetic fields. This part of the portfolio relies on the well-established technologies provided through CST Studio Suite and Opera.
Charged particle dynamics simulation is essential to analyzing and optimizing various charged particle devices. The simulation process of a particle's life can start with the emission of the particles and the effects of accelerating electrostatic and focusing magnetostatic fields that they are exposed to. In addition, these devices creating external fields are carefully designed using highly accurate static simulation. At very high energies, the relativistic equations of motion must also be considered.
Particle simulation can consider the fields generated by the particles as space charge, which overlays the external electromagnetic fields. The self-electromagnetic fields can introduce a transient component that acts back on the particles. At this point, we require a fully self-consistent Particle-in-Cell simulation.
To reach higher particle energies, the particle beam is exposed to RF-fields. An electron beam can now approach the speed of light, the ultrarelativistic limit. The particle beam is considered as a current that creates electromagnetic fields, wake fields that can act back on itself or on following beams. Various beam optic devices guide the beam.
CST Studio Suite and Opera include several tools for designing charged particle devices. Besides the typical static and high-frequency solvers, there is the Particle Tracking Solver, the Electrostatic Particle-in-Cell (Es-PIC) and the standard Particle-in-Cell (PIC) Solver and the Wakefield Solver. These are used to design beamline components from particle sources, to magnets, to cavities, to absorbers.
Particle dynamics simulation is also crucial in the design of vacuum electronics devices. Magnetrons, gyrotrons, klystrons and traveling wave tube amplifiers are among the components that can be designed with CST Studio Suite. Breakdown effects such as multipaction and corona effects can be simulated and, with multiphysics simulation, the thermal and mechanical effects of high-power microwaves can also be taken into account.
Particle Dynamics Applications
- Particle Accelerators
- Particle Sources and Electron Guns
- Vacuum Electron Devices
- Plasma
- Multipactor and Corona
- Magnetron Sputtering
Particle Accelerators
Accelerator components
Accelerator components such as cavities or beam position monitors are typically designed with the Eigenmode, Transient or Frequency Domain solvers. However, for the interaction with the beam, the Wakefield solver is an incredibly versatile tool. You can read more about CST Studio Suite solvers here.
A 9-cell cavity of the TESLA accelerator is shown here. The goal is to maintain the electron beam acceleration during the whole propagation of the beam along the accelerator. Radiofrequency (RF) power is generated and coupled into the TESLA cavities to establish these EM fields in the cavities. The electrons passing through the cavities must keep the right phase in relation to the field to maintain acceleration. The electron beam, a strong current by itself, induces high-frequency fields and excites modes, the so-called wake fields while traveling through the cavities. These wake fields could limit or interrupt the acceleration process. The Wakefield solver computes these fields and helps to improve the design of the accelerator components.
Beam Optics
Particle accelerators use magnets and electrodes to direct, refine and control the particle beam. Typical beam optics components include magnetic and electrostatic lenses to focus the beam, deflectors to bend and steer the beam, kicker magnets to redirect the beam, and collimators and collectors to safely capture the particles.
The SIMULIA tools Opera and CST Studio Suite have been used to successfully design all types of magnets for accelerators: permanent magnets, DC and AC dipoles, quadrupoles, and higher-order magnets, undulators and solenoids. Particle tracking solvers simulate the motion of particles through the simulated fields, with or without space charge effects.
Opera can simulate both low and high temperature superconductors, including superconducting quench events where a superconducting magnet rapidly transitions to the normal state. It is possible to include multiple species of charged particles, each having user-defined charge and mass.
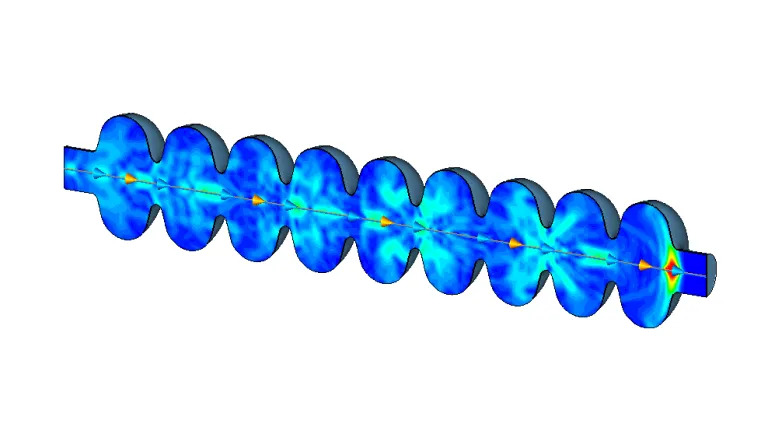
Particle Source and Electron Gun Simulation
Electron Guns
Electron guns are the sources of particles in many industrial, medical and research applications, from X-ray tubes to traveling wave tube amplifiers. These often require refined beams with limited dispersion. However, the space charge repulsion between electrons means that the beam tends to diverge without careful design of the electrodes.
Particle tracking solvers in CST Studio Suite and Opera can model the movement of electrons through the electric field inside the gun. Space charge models simulate the repulsion between electrons and the resulting beam dispersion, allowing engineers to accurately simulate beam behavior and produce a reliable beam. Comprehensive multi-physics simulation can investigate thermal and stress in addition to electromagnetics.
Carbon nanotube tube emitters
Carbon nanotube tube emitters produce electrons at room temperature (cold cathode) by the quantum mechanical field effect, which requires less electrical power. Because of their size, these can be used in more portable devices. Opera allows the development of these increasingly popular electron sources.
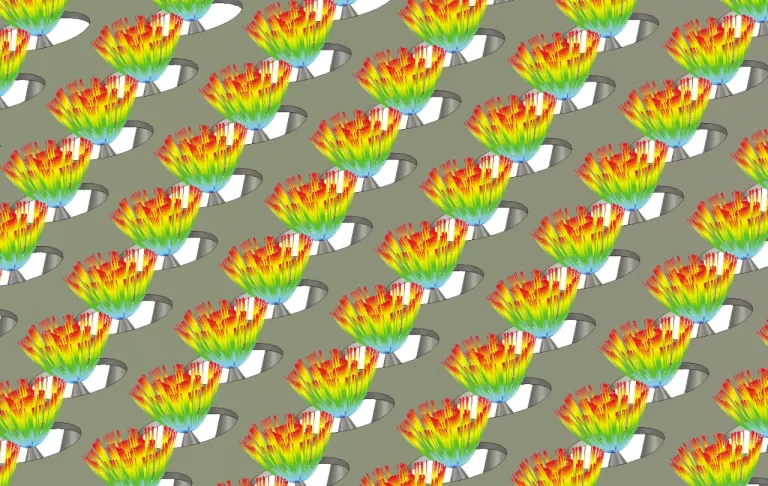
Vacuum Electron Devices
Vacuum Electron Devices such as Traveling Wave Tubes (TWT) are used mainly for satellite communication because of their reliability and performance. For instance, in the frequency range between 1-60 GHz, the amplified signal can reach an output power up to 500 W with an efficiency over 50% (for space TWTs).
In contrast to their solid-state counterparts, they show higher efficiency, higher reliability, better thermal performance and a slightly better linearity. However, they are more expensive to build. Thus, TWTs are used when reliability is a must, such as for high powers and on satellites. Simulation is attractive in these design processes as it reduces the need for multiple costly prototypes.
The design of a TWT can be performed using the PIC solver to characterize the Slow Wave Structure (SWS) which corresponds to the interaction region between the electron beam and the RF signal sustained by the helical structure.
An RF signal is introduced from an input coupler. During the propagation of the electrons along the SWS, the kinetic energy of the electrons is transferred to the traveling wave. Along the tube, the electron beam becomes bunched and the electrons lose their kinetic energy to the traveling wave. The traveling wave is then amplified with a maximum power extracted in the output coupler.
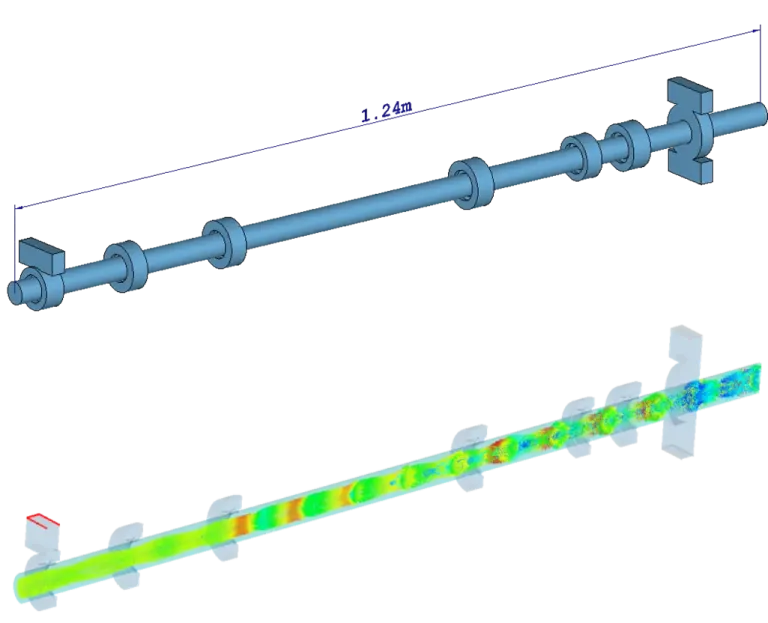
Plasma Simulation
Plasma Applications
Plasma applications typically have large time scales and the plasma can be described by its space charge interaction between the electrons and the ions. The Electrostatic Particle-In-Cell (ES-PIC) technology computes space charge versus time, taking into account the electrostatic effect only. Compared to a pure PIC approach, there is no current and H-Field induced, but it is very well suited for these plasma applications. It also stays valid for plasma applications where the phenomenon can be described by the space charge dynamics and collisions at relatively low pressure, neglecting the temperature gradients of the ions and the convection effects, which would require another numerical approach.
Power Sources for Fusion Plasma
Plasmas for fusion are very hot. They are created in tokamaks and provide a new source of energy production. Fusion power is one of the sustainable energy sources under investigation today to answer the energy problems the world is facing. The energy of the future must come from clean, safe and controlled fusion.
In the main operating mode, the plasma must be confined. This is the role of the complex magnetic coils design surrounding the tokamak. Then, the plasma must be sufficiently hot to maintain the thermonuclear reactions. This is the role of the Gyrotron devices, which can be fully designed and simulated with the PIC solver.
Gyrotrons are high-power vacuum electron devices capable of generating output powers in the order of hundreds of kW with operating frequencies up to several hundreds of GHz. Gyrotrons are well suited for plasma heating process because the microwave frequency generated can excite one of the plasma frequencies. The waves transfer their energy to the plasma, leading to the heating process.
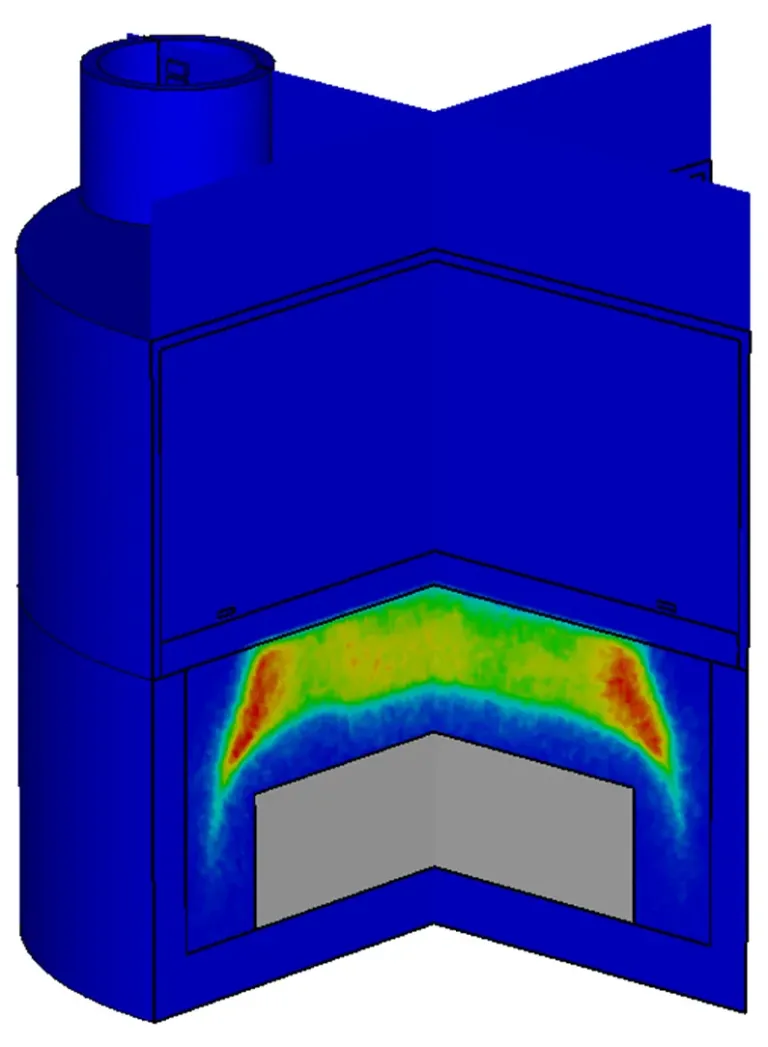
Multipactor and Corona Simulation
SIMULIA offers special RF breakdown analysis such as Multipactor and Corona effects based on Spark3D technology. These effects occur in high-power microwave components and can destroy sensitive devices. Multipactor is a major concern for satellite communications, where a component failure can render the whole system inoperative.
Two types of investigation can be performed here. When working at atmospheric pressures, the Corona effect is dominant whereas for components embedded in space, which means very close to vacuum, the Multipactor effect is dominant. The multipactor is governed by the material properties and the so-called Secondary Emission Yield – the probability of an electron collision causing the emission of another electron. Multipactor occurs when the power of the RF device is strong enough that electrons are accelerated leading to a multiplication of secondary electron emission, creating an avalanche of electrons.
Multipactor and Corona effects are strong constraints that must be considered during the design phase of the RF-components and the qualification tests.
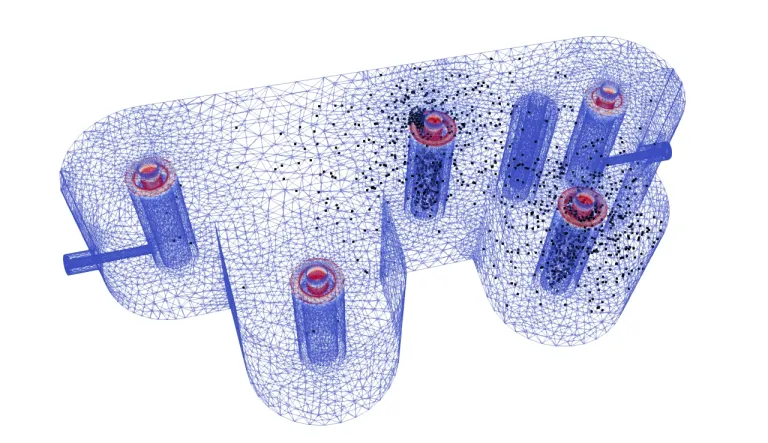
Magnetron Sputtering Simulation
Sputter coating is widely used for the fabrication of thin films in a highly diverse range of areas. Applications include decorative and low emissivity coatings on glass, through to engineering coatings on products used in today’s most demanding applications. Optimization of the deposited film properties and utilization of the sputter target are critical for the performance of the end-product and for the economics of the process. SIMULIA Opera combines accurate finite element analysis with detailed models for plasma, sputtering, and film deposition to provide the first practical tools for magnetron design and optimization.
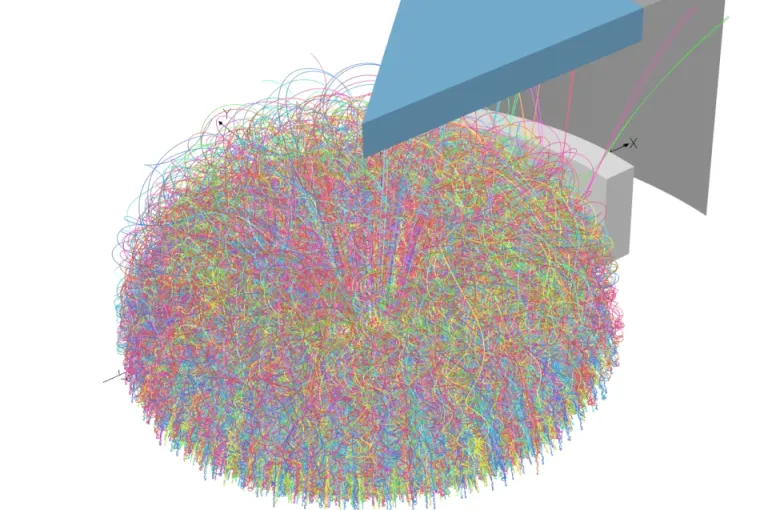
Start Your Journey
Explore the technological advancements, innovative methodologies, and evolving industry demands that are reshaping the world of Particle Dynamics Simulation. Stay a step ahead with SIMULIA. Discover now.
FAQs on Charged Particle Dynamics Simulation
A particle traveling through a magnetic field is subjected to the Lorentz force. The Lorentz force acts perpendicularly to the direction of motion of the particle. It does not change the velocity or energy of the particle; however, as a centripetal force, it introduces a circular motion.
Charged particles are typically created in dedicated particle sources such as electron or ion guns. Commonly found guns use thermionic emission to generate a particle beam. Built from special materials, they emit electrons when sufficiently heated, which are accelerated towards an anode. Well-known examples are cathode ray tubes.
Also Discover
Learn What SIMULIA Can Do for You
Speak with a SIMULIA expert to learn how our solutions enable seamless collaboration and sustainable innovation at organizations of every size.
Get Started
Courses and classes are available for students, academia, professionals and companies. Find the right SIMULIA training for you.
Get Help
Find information on software & hardware certification, software downloads, user documentation, support contact and services offering